Dongwha Lee
Reducing carbon dioxide (CO2) emission into the atmosphere is one of the most important challenges, society needs to address in this century. The development of new technologies that are both economically viable and technologically efficient is a key research goal in recent years. The question of how the CO2 emission could be controlled is closely connected to progresses in different technological avenues pursued by the scientific community and industry (e.g. geological sequestration and photocatalytic reduction, etc). Each approach has both advantages and disadvantages and a combination of multiple approaches might be required to address the CO2 emission problem as a whole. Our group has been using two different approaches to address this issue.
1. Biomimetic CO2 hydrolysis
Hydrolysis of carbon dioxide occurs rapidly by enzyme inside the human body. Diverse studies have proven that the CO2 hydrolysis can be mimicked into much small molecular system with a metal-porphyrin active site. In this study, we have implemented the active site into carbon nanotube (CNT). Using accurate first principles electronic structure calculations, we predict how the catalytic hydrolysis reaction in carbonic anhydrase (CA) can be mimicked in a metal-porphyrin CNT. The two-step catalytic process can be improved remarkably by controlling the porphyrin oxidation state via the nanotube charge state and by substituting the porphyrin metal atom. The oxidation state and the metal substitution both have profound effects on the reaction energetics for the initial hydration reaction step. For the subsequent product-release reaction step, two different reaction mechanisms could take place. These mechanisms are distinctively sensitive to either the oxidation state change or the metal substitution but not to both. For the overall catalytic cycle, a significant dependence on the nanotube charge state at low pH and on the metal substitution at high pH is expected.
Figure: Schematic of the catalytic cycle (left) in carbonic anhydrase (CA) is shown. The zinc cation is surrounded by three imidazole ligands (Im). The hydroxyl group reacts with CO2 molecule at the transition metal site, to form zinc-bound bicarbonate. The bicarbonate then reacts with either a proton or a water molecule, releasing the molecule from the active site of CA and to regenerate the zinc hydroxide. The structure of zinc-porphyrin CNT (right top) is drawn with the electron rich (blue) and deficient (gray) region near the porphyrin under different oxidized condition. Potential energy profile (right down) along the normalized reaction coordinate for CO2 hydration reaction step is shown under different oxidized condition. The potential energy profiles in different charge states are shown with respect to the reference charge state [1].
[1] Donghwa Lee, and Yosuke Kanai, "Biomimetic Carbon Nanotube for Catalytic CO2 Hydrolysis: First-Principles Investigation on the Role of Oxidation State and Metal Substitution in Porphyrin", The Journal of Physical Chemistry Letters 3, 1369-1373 (2012)
2. Photo-catalytic CO2 to fuel conversion
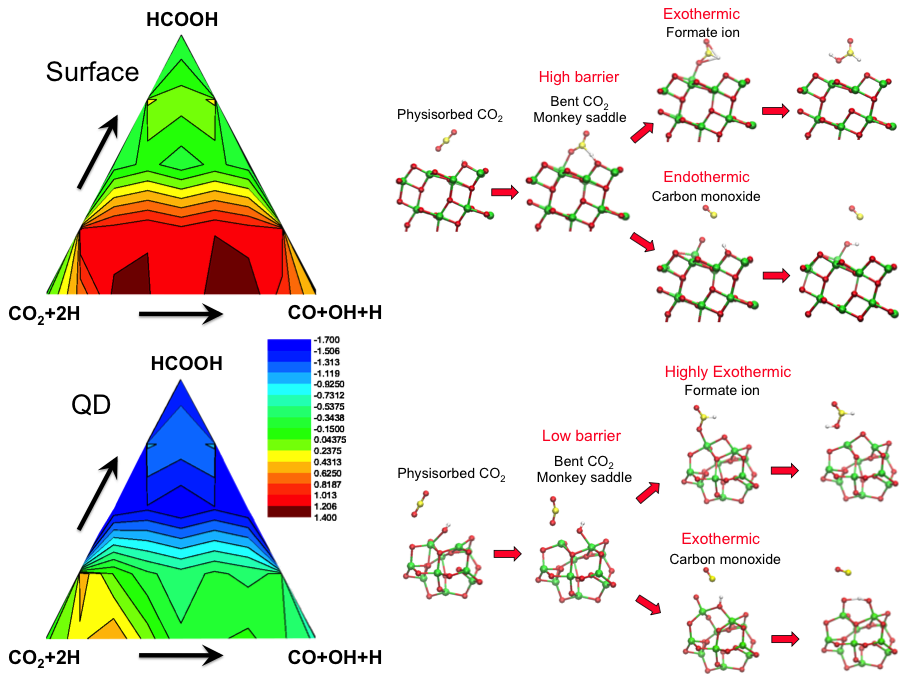